IgG4, COVID, and mRNA vaccines: Not so Tolerant
mRNA vaccines produce an unusually high fraction of IgG4 against spike. Some have falsely claimed this indicates tolerance developing against SARS-CoV-2. The truth of IgG4 is more complex.
A while ago, a finding was published showing that after the booster dose of mRNA vaccines, there was a dramatic expansion in IgG4 antibodies against the spike protein. This caused some people very much undue alarm. At the time, I tried to address the panic with a bit of context, but unfortunately it continues to be distorted, although now in a different way than I initially addressed. In short, IgG4 does not automatically indicate tolerance to SARS-CoV-2 (and this position is not supportable with the available data). Whether or not an IgG4 response against the spike protein is worse than a non-IgG4 response is not entirely clear and not at all a simple question to answer. Still: we do not bring a mechanism to an epidemiology fight1, meaning if we want to know whether or not COVID vaccines are protective, we should prioritize looking at a study of their effectiveness over fixating on mechanisms. The mRNA vaccines are strongly protective, as evidenced by clinical trial data and real world effectiveness studies, regardless of what the specific role of IgG4 is.
We do not bring a mechanism to an epidemiology fight.
What is IgG4?
IgG4 is a subclass of antibody. Antibodies are Y-shaped proteins made by antibody-secreting cells, which come from B cells. They might also be called immunoglobulins, leading to the abbreviation “Ig.” Each antibody is made of two proteins (a heavy chain and a light chain) which are linked together. Each heavy chain and light chain in turn has a constant and a variable region. Antibodies recognize antigens and the specific part of the antigen recognized by an antibody is called an epitope. The epitope is bound by the antibody’s paratope:
Humans are capable of making roughly a quintillion (1,000,000,000,000,000,000) distinct antibodies. These antibodies differ from each other in a number of ways. One is binding specificity (which antigens, or rather epitopes, the antibodies bind), but in addition to this, antibodies can have different classes or isotypes. These are defined by the constant region in the heavy chain of the antibody. Humans have 5 classes of antibodies: M, D, G, E, and A (sometimes written with their Greek letters- μ, δ, α, γ, ε; the light chain also has 2 classes to pick from -κ and λ- but these are not known to have functional importance2). The A and G classes have subclasses. A has 2 (A1 and A2) and G has 4 (G1-G4). Hence, an antibody with the G4 class can be abbreviated IgG4. Different antibody classes and subclasses will have different properties in the immune system even if they bind the exact same target antigen because they differ in their ability to bind Fc receptors (proteins that recognize the “stalk” part of the antibody). These Fc receptors control the effector functions of antibodies (i.e. what responses they elicit from cells that have Fc receptors). However, neutralization, the ability of an antibody to stick to something and deactivate it (like a toxin or a virus), is determined by the Fab region- specifically what part of the antigen is being bound and how tightly (however, Fc effector functions can support neutralization). The way an antibody interacts with Fc receptors is the most important factor in how that antibody behaves in the body.
In the course of an immune response, antibodies originally all begin as IgM, and then undergo a process called class switch recombination (CSR) in which DNA in the B cell (which encodes the antibody heavy chain sequence) is looped out and excised, removing certain antibody classes and forcing the use of other ones. This is similar to highlighting text in a word document and deleting it:
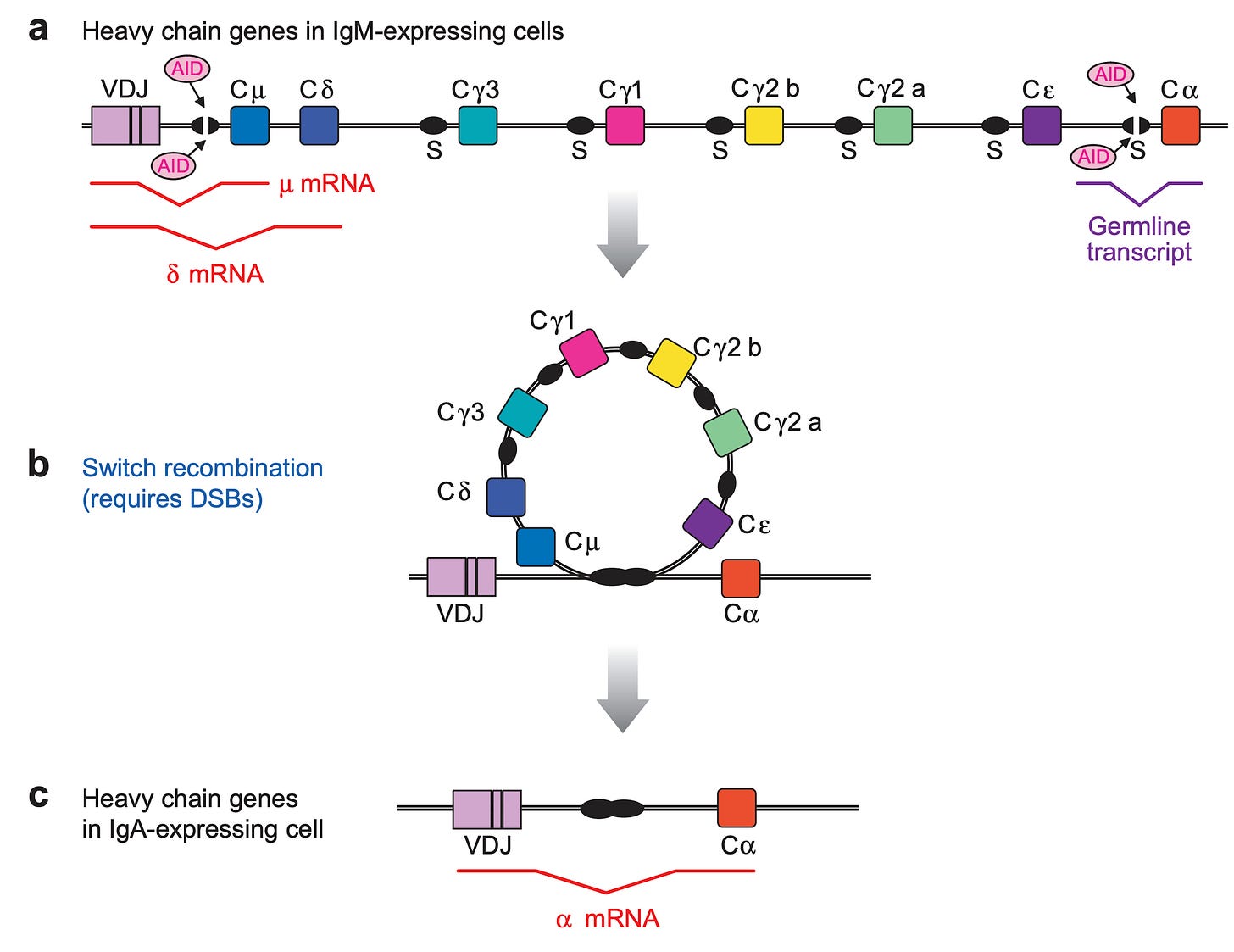
The class that an antibody is switched to is determined by the cytokines (small proteins made by immune cells that tell other immune cells what to do), as well as certain other immunologically important molecules, which in turn are determined by the type of threat. If interested, details on the control of class switching can be found in this review, but for this discussion it will be enough to just know class switching exists and is a fundamental property of the immune system controlled by (mainly) cytokines.
Next I present a few tables and images- the details are not important, but they illustrate the previous points more concretely. This table gives a (very abridged) summary of how different cytokines direct different antibody class switching in humans:
The cytokine produced in turn will depend on the type of threat confronted, as described here:
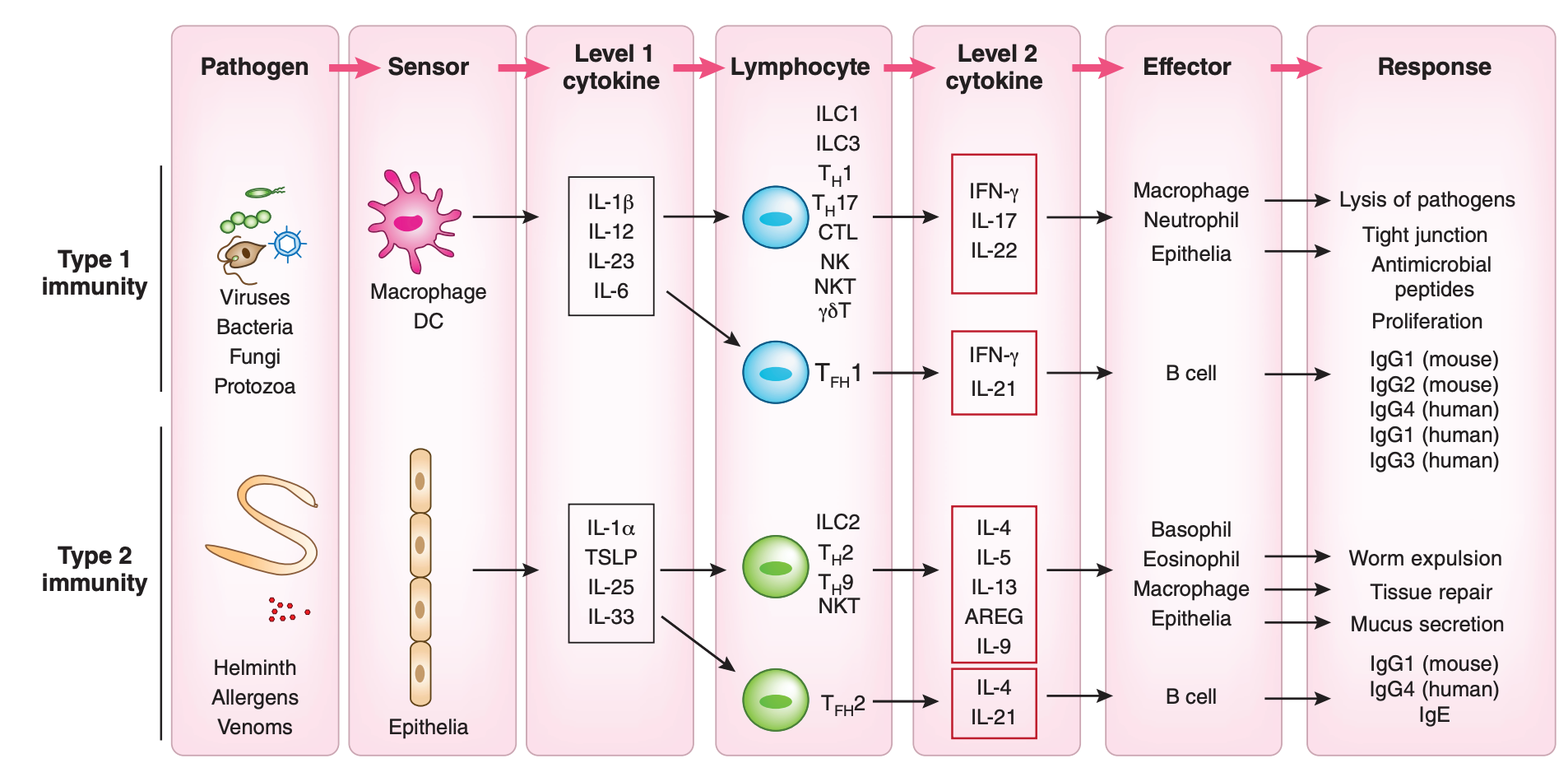
For example, viruses all but universally induce the production of type 1 interferons (like IFN-α and IFN-β), which as per the table inhibits the switching to IgE; IgE is generally not going to be useful in dealing with a virus, as its major function is to activate mast cells. In contrast, infection with parasitic worms (like tapeworms) will readily induce IL-13, which in turn will promote switching to IgE; this results in functions that promote the removal of the worm from the body (worms are generally too large for our immune system to kill them so instead the body tries to throw them out; for example, activating mast cells also promotes contraction of smooth muscle, such as that in the intestine, which can help expel worms from the body). This offers the immune system a way to tune the type of response to the threat it encounters.
Class switching can occur just once in a given B cell clone or multiple times, in which case it is known as sequential class switching3. However, for sequential class switching to be possible, you need to have the DNA sequence for that class present.4 For example, if you have already made IgE, you cannot class switch to IgG4 because to make IgE, you must excise the DNA that contains the IgG4 constant region. However, if you make IgG4, you can still class switch into IgA2, because the IgA2 constant region occurs after IgG4 in humans, and indeed this is thought to occur in humans.
The Function of IgG4
IgG4 is the least abundant IgG subclass in the blood, with about 3-6% of all IgG in the plasma being IgG4 under normal conditions (but the specific number you get will vary from person-to-person and reference you consult; IgG1 is usually about 75%, for reference). The specific requirements for eliciting IgG4 are incompletely understood, but we do know a lot. IgG4 is generally seen in instances of “hyperimmunization,” meaning prolonged, high-levels of exposure to an antigen, emerging late in the course of the response (emphasizing the chronic nature of the exposure). An example of this is allergen immunotherapy in which people are repeatedly dosed with an allergen frequently (e.g. multiple times per week, initially). The intention here is to develop IgG antibodies (including IgG4, but usually IgG1 initially) as these will compete with the IgE antibodies for binding to the allergen but avoid activating mast cells5. The IgG1 and IgG4 here are induced by new responses to the allergen, not sequential class switching. IgE requires binding to its allergen to activate mast cells and cause the allergic reaction. The appearance of IgG4 against the allergen is often a sign that desensitization to the allergen has been successful.
IgG4 is also known for a set of autoimmune conditions called IgG4-related disease. These conditions are aptly named, as the emphasis here should be on the “related” rather than the IgG4. The conditions share in common that they involve a proliferation of IgG4-secreting cells, but they appear to be driven by an unusual population of T cells which drive both inflammation and fibrosis (i.e. scarring) which are stimulated by the IgG4-secreting cells through the latter’s presentation of antigen:
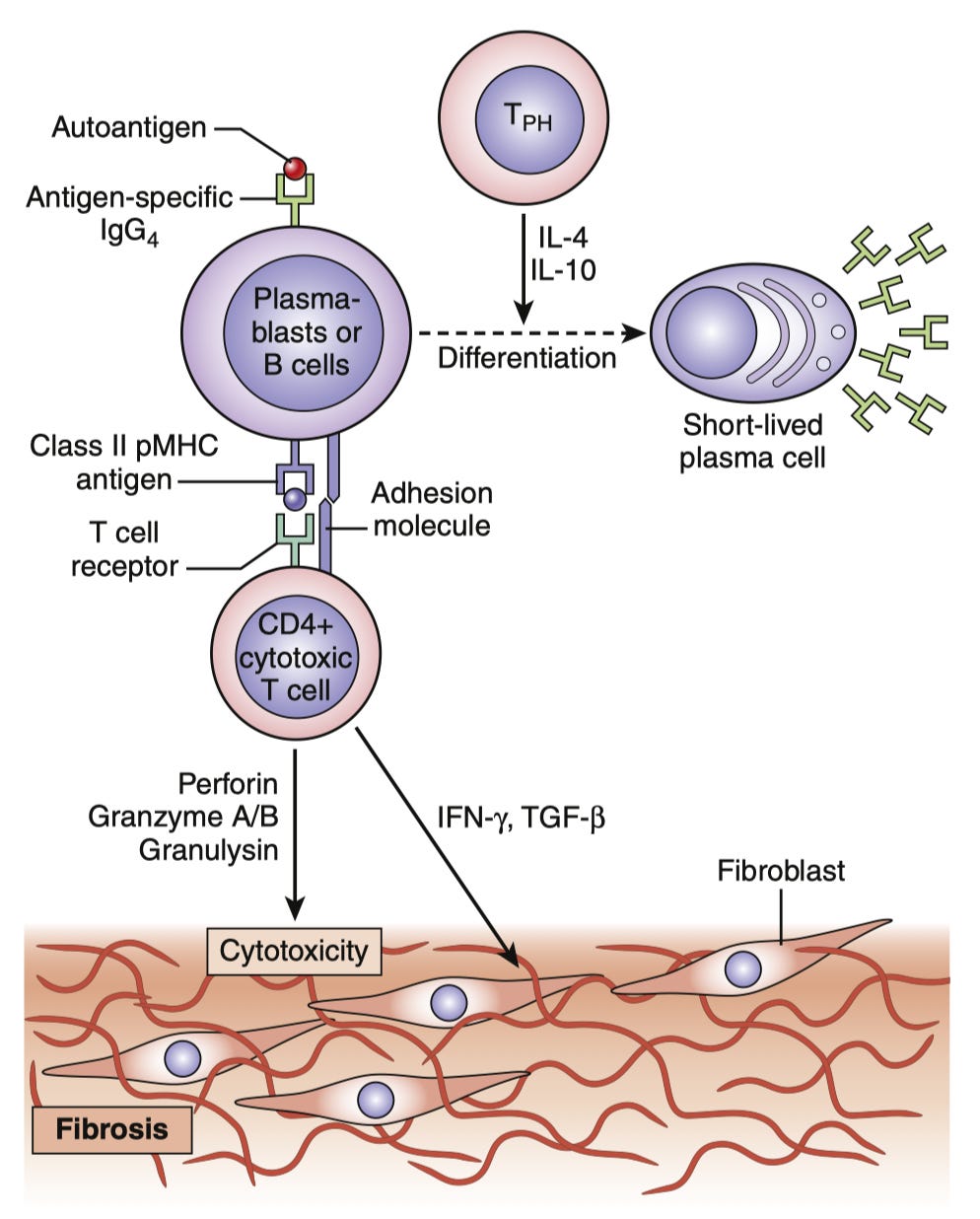
Aside from its rarity, IgG4 has several important functional differences from the other subclasses. The most prominent of these is known as Fab arm exchange. The IgG molecules all have 2 Fabs. However, the bonds that hold together the two heavy chains of each IgG are much more chemically fragile in IgG4, giving it the ability to form so-called halfmers, wherein it has only 1 binding site. Alternatively, IgG4 halfmers can recombine with one another to form IgG4s that have different binding specificities:
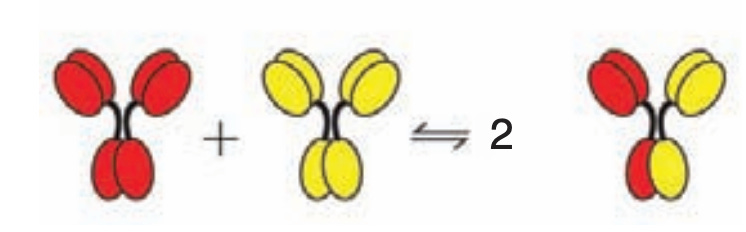
In addition to binding, antibodies exert many effects on the immune system through proteins called Fc gamma (Fcγ because they bind IgG) receptors which sense the Fc regions of antibodies (recall that these differ between classes and subclasses of antibody).
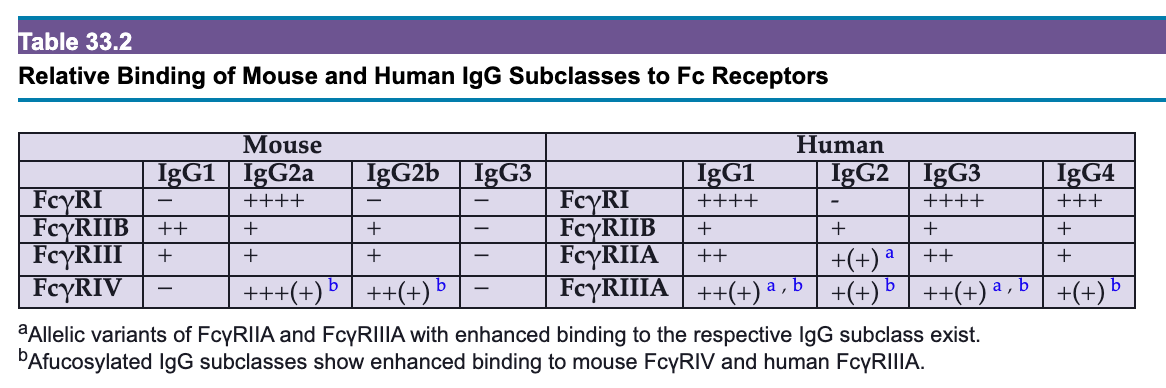
Each of these receptors6 binds one IgG at a time, and a meaningful signal to the cell will not occur unless multiple receptors are activated simultaneously7. Thus, signaling generally only occurs in the context of antibody-antigen lattices called immune complexes as these allow for crosslinking of Fc receptors, which also depends on the relative quantities of antibody and antigen:
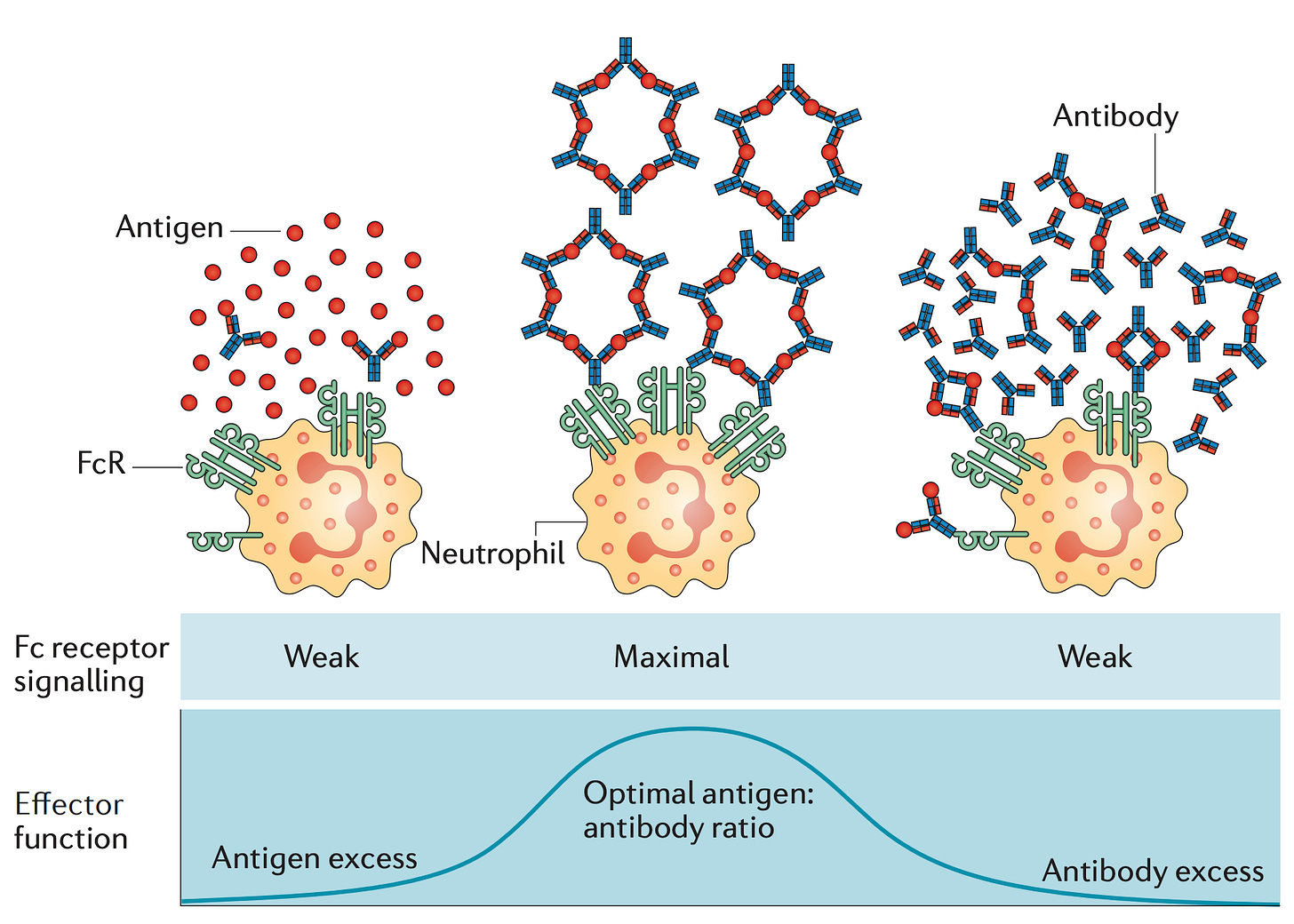
If Fab arm exchange occurs, the ability of IgG4 to form immune complexes (and consequently signal to immune cells) is drastically reduced because IgG4 loses the ability to cross-link antigens (bind 2 antigens at the same time).8 Still, if enough IgG4 is present and the antigen has multiple binding sites for the IgG4, immune complexes will still form. In addition to this, the IgG4 Fc region has unique structural features that reduce binding to Fcγ receptors.
This has led to some thinking that IgG4 arises specifically to tone down overactive immune responses to limit tissue damage (a function that, as I will explain shortly, is absolutely critical in the setting of some infectious diseases). Still, it’s important to be careful about oversimplifying. While IgG4 is less capable of forming immune complexes, its ability to do this is not absent. Additionally, it is important to remember that while subclass is an important modifier of antibody function, it is not the only modifier. Antibodies are elaborately adorned with different sugars (glycans), and differences in the arrangement and choice of these glycans has profound consequences. For example, the absence of a sugar called fucose, producing afucosylated antibodies, drastically enhances binding and signaling through FcγR3A- and this holds true even for IgG4. Thus it is important to evaluate these effector functions of antibodies empirically rather than just looking at subclass composition. Additionally, IgG4 antibodies tend to have extremely potent neutralizing ability when it comes to their target antigens, including viruses.
Where does IgG4 from mRNA vaccines come from?
First, it should be stated that the appearance of IgG4 itself in response to mRNA vaccines is not unusual, as IgG4 shows up in response to other vaccines and infections. The unusual aspect is the proportion of antibodies that end up being IgG4:
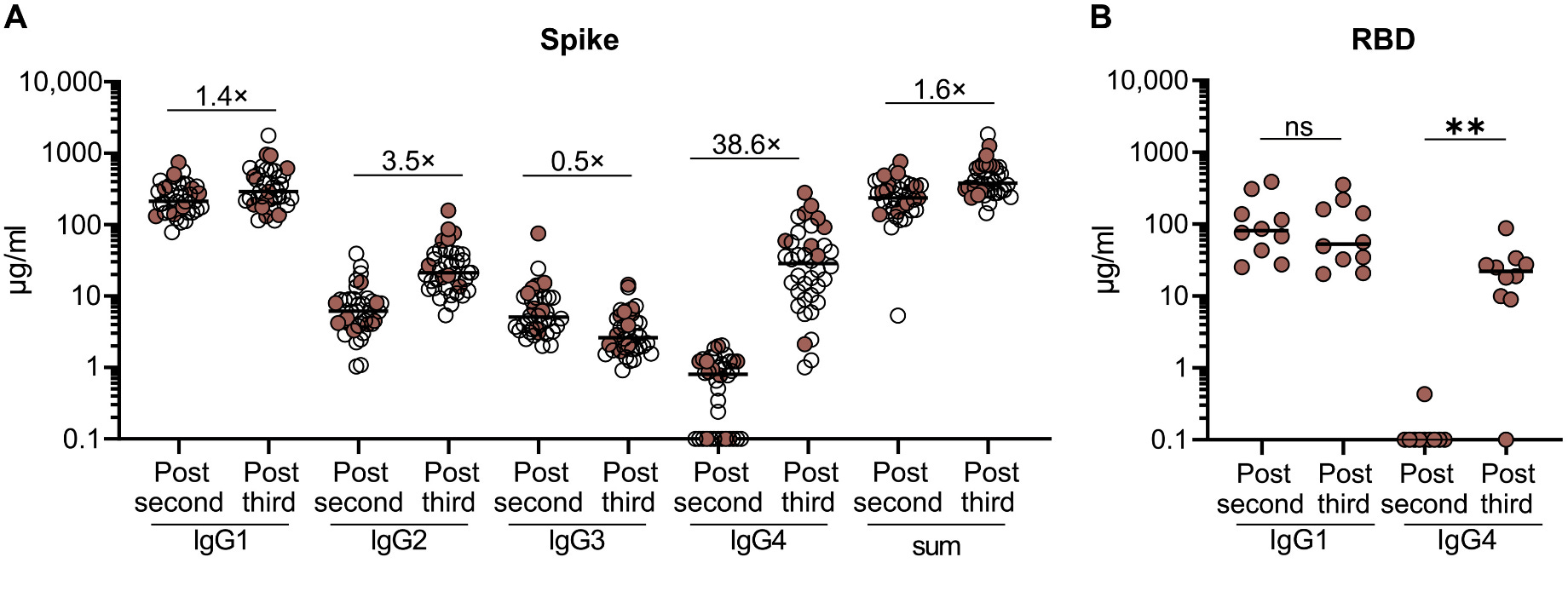
After the third dose, the quantity of IgG4 against spike increases by nearly 40-fold, and it ranges from being 40-80% of the total anti-spike IgG. Still, in most people, most of the IgG is IgG1. Additionally, this IgG4 is not coming from the third dose as some people have erroneously described- this is coming from a recall response from the second dose. IgG4 is being made late in response to the second dose of the mRNA vaccines (presumably because the immune response to the mRNA vaccines in the lymph nodes, the germinal center reaction, is so long-lived), which is demonstrated by the existence of many IgG4 memory B cells BEFORE the third dose is given. This means that even if a person were to foolishly decide that this finding means they should not get the booster, they would still generate an IgG4 response against SARS-CoV-2’s spike when they were infected (in fact, in this study, this exact thing happened to a few people and the IgG4 response was GREATER in response to COVID-19 than to the booster). This is also clearly dependent on the priming series- the switch to IgG4 is not seen in those who initially received adenovirus-vectored vaccines. It is also noteworthy that among the breakthrough infections, those who had one more than 70 days after the second dose showed the pattern of IgG4 switching, but those who were infected before did not.
The authors propose, consistent with what is known about IgG4, that this represents a way to tone down the inflammatory response in the lymph nodes being driven by other subclasses of spike antibody, and that the delivery of the two doses of mRNA vaccines together in such close proximity (3-4 weeks) is a key factor in this response. This is a compelling idea, and while there are data on the effect of longer intervals between doses of the mRNA vaccines on the immune response, I am not aware of any that have looked specifically at this aspect of it.
More information has recently come to light however regarding what could be driving this phenomenon. It has been noted that those who take dupilumab (an antibody against the IL-4 and IL-13 receptor that blocks these cytokines’ effect) or tumor necrosis factor (another cytokine) inhibitors do not exhibit this switch to IgG4 upon mRNA vaccination. This indicates that these cytokines are directly involved in the class switch to IgG4 from mRNA vaccines. This also offers an opportunity to examine whether the IgG4 vs other IgG subclasses specifically contributes to better or worse outcomes in COVID-19, but some caution is warranted. Specifically, dupilumab is associated with better outcomes against COVID irrespective of vaccination, which may skew results, and it has effects on the immune response beyond just antibody subclass.
Does IgG4 indicate tolerance?
Immunological tolerance is the ability of the immune system to, essentially, not react to the presence of something in the body (i.e. it tolerates that it is there). An example of this would be self-tolerance, where the immune system does not respond to substances found in our own cells (a process that breaks down in autoimmune disease, wherein our immune system recognizes self-antigens as threats). IgG4 is often described as an anti-inflammatory or tolerogenic (i.e. induces tolerance) antibody, which is an oversimplification. In some contexts, IgG4 is clearly tolerogenic9, such as when it is induced against an allergen. Yet, this is also true of the IgG1 induced early on in response to the allergen, but people do not generally describe IgG1 as being tolerogenic. IgG4 is probably best described as non-inflammatory, or, even more properly, less inflammatory than other subclasses. However, IgG4 can still exhibit the same effector functions as any other IgG subclass (but is less efficient at them), and IgG4 shows functional changes similar to other subclasses in response to changes in its glycan.
The idea that vaccinated individuals are developing tolerance to SARS-CoV-2 is not supportable in any data I have seen, which would likely have to demonstrate an increased risk of chronic infection by SARS-CoV-2 in vaccinated vs. unvaccinated individuals. The fact that vaccination is so consistently shown to improve outcomes and people do not (in general) test positive indefinitely argues strongly against this.
Is the fact that IgG4 is elicited by mRNA vaccines bad for people who got them?
This is big question that many people have asked me, which is basically their way of asking whether or not it was a mistake to get an mRNA vaccine or a booster. However, these are two very different questions. Let’s start with the one that people actually mean: no, it was not a mistake to get an mRNA vaccine nor a booster. The data are absolutely overwhelming in that mRNA vaccines drastically reduce the risks of COVID-19 in every single demographic they are given10. While there are rare but real risks to them and some people commonly feel quite crummy for a day or two after getting them, they remain extremely safe and very effective. Choosing to face COVID without them would have meant a catastrophic and unnecessary loss of life. This is not intended to negate the critical contributions of other vaccines against COVID-19 which were also clearly lifesaving, but of all vaccine classes for which we have data, mRNA vaccines were clearly the most effective in head-to-head comparisons.
Whether or not IgG4 itself is a problem with mRNA vaccines remains to be resolved, and a reasonable argument can be made either way. Even though neutralization markedly improves after the third dose of vaccine, it is possible that it would be better for antibody subclasses that do not perform Fab arm exchange. For example, IgG3 has a very flexible hinge region that allows it to better crosslink antigen, meaning it can achieve better neutralization with the same paratope as other subclasses. It is, however, noteworthy that in this study IgG1 and IgG2 showed similar neutralization to IgG4. It is also shown that optimal protection from antibodies against SARS-CoV-2 (unsurprisingly) requires both neutralization and Fc effector functions. Still, while these things are true, it is not as though the Fc effector functions of the serum vanish with the switch to IgG4. The major function that is shown to decline with the shift to IgG4 is complement deposition11; however the role of complement in protection from COVID-19 (and viruses generally) is not at all clear, and in fact complement is associated with the devastating clotting conditions that occur in COVID.
There are several lines of evidence that suggest that the reduced propensity of IgG4 to elicit inflammation is strongly protective in COVID-19. I should take a moment to disabuse of a misperception though: the key to a successful immune response is not just to clear the threat as quickly as possible but to do so with as little tissue damage as possible. In some cases this is very extreme. For example, Dengue causes a state of massive leakage from the vasculature driven precisely by afucosylated antibodies and FcγR3A, in part through the unique ability of dengue to take advantage of antibody-dependent enhancement (evidence for which is still lacking with SARS-CoV-2). In another example, respiratory syncytial virus causes such havoc also through excessive immune responses, and a vaccine formulation designed to induce regulatory T cells (a subset of T cells that actively suppresses inflammation) in response to RSV showed strong protection in animals.
Excessive activation of FcγR3A is a major (possible one of the most important) drivers of hospitalization in COVID-19, and IgG3 has the highest affinity for this receptor of all of the subclasses. Indeed, IgG3 has 13 genetic variants (allotypes), most of which shorten its half life to 7 days (vs ~3-4 weeks for the other IgG subclasses), which is thought to have evolved because giving IgG3 the standard half-life may result in excessive inflammation. In the same vein, despite equivalent ability to neutralize virus, individuals who received the ChAdOx vaccine who demonstrated an antibody profile that promotes inflammation (with IgG3) were more likely to develop COVID than those who were able to eliminate virus in an immunologically silent manner. This argues against IgG4 being a problem.
Lastly, we should bear in mind that the antibody response is just one component of the entire immune response to COVID-19. One of the first cells to respond to SARS-CoV-2 infection is actually the humble CD8 T cell, and the CD8 T cells elicited by the mRNA vaccines (as well as the adenovirus vectored vaccines) do damn fine work. In summary: I think it’s perfectly reasonable to wonder about what the exact significance of IgG4 from the mRNA vaccines is, but people should take care not to confuse this with other questions. So please: stop bringing mechanisms to an epidemiology fight.
I use this expression to mean that whenever it is possible to look for an answer to your question directly or to understand the significance of a given phenomenon, that is always going to be preferable to reasoning mechanistically. Still, there is a prominent exception to this rule that I would be remiss not to raise.
In some circumstances (like a public health emergency, or at least a situation in which there are major time constraints on getting the data), answering a question epidemiologically may take too long to be able to capitalize on the benefits. A classic example of this is with seasonal updates to COVID and flu vaccines. If we were to have to run a full-scale efficacy trial on every update, the variants we would target with these vaccines would no longer be there and we would have forfeited considerable ground. Instead we have a very strong reasonable surrogate: neutralizing antibody titer. Why is this surrogate okay?
We have data showing that neutralizing antibody levels correlate with protection from infection and mortality in COVID-19
We have (had) monoclonal antibodies that worked to protect from COVID by neutralization (until variants escaped them)
We have animal studies showing that antibodies alone are enough to be protective against COVID-19
Antibody response is associated with the function of a number of other immunological responses that are critical to protection from COVID
We have similar evidence for all of the aforementioned with influenza as well
We do not have a threshold at which we can say a vaccine is likely to be protective vs. not (this is formally called an absolute correlate, but people often refer to this as a correlate of protection), but it is an entirely reasonable assumption that something that enhances the antibody response substantially against influenza or SARS-CoV-2 will enhance protection barring some kind of drastic change to the virus. Furthermore, when we’re talking about a minor change (like, a few amino acids) to a vaccine that we have given billions of doses of, whose safety profile is very well understood, the idea that changing out a few amino acids will cause some dramatic change is just not parsimonious.
It has also been suggested that our immunogenicity studies are too antibody-centric by some experts, which is a reasonable criticism, but this does not negate the data we have supporting the value of antibodies as surrogates.
This is not to say that we do not need effectiveness studies of COVID or flu vaccines ever -we need these data- but sometimes we have to make do with less than perfect methods to maximize the benefit of our interventions. So long as those benefits are being maximized, that is a reasonable price to pay.
While κ and λ are not known to be functionally distinct, you can gain useful information from them. For example, in humans the normal ratio of free κ:λ light chains is between 0.26 to 1.65. If there is a significant shift in the ratio, it suggests excessive proliferation of a specific B cell clone (numbers less than 0.26 suggest it uses the λ light chain, and numbers above 1.65 suggest it uses κ), as may be seen in B cell cancers like multiple myeloma. It should be noted however that while this information may be helpful, particularly for monitoring the progression of these conditions, the ratio of light chains on their own cannot diagnose a B cell cancer.
The major reason sequential class switching is relevant is that it is thought to be a major way in which people develop allergic IgE antibodies. Specifically, it is thought that much IgE arises from sequential class switching from an IgG1 memory B cell which has already undergone affinity maturation. This produces IgE with very high affinity to the allergen, which is responsible for allergic reactions, including anaphylaxis. However, recent findings indicate that sequential class switching is not required.
Theoretically, there is one exception to this. Because we have two copies of all of our DNA (one from mom and one from dad), it is theoretically possible to have recombination between regions that are very similar (known as homologous recombination). The mechanism is a bit complex but can be summarized as follows (from Watson’s Molecular Biology of the Gene, 7th Edition):
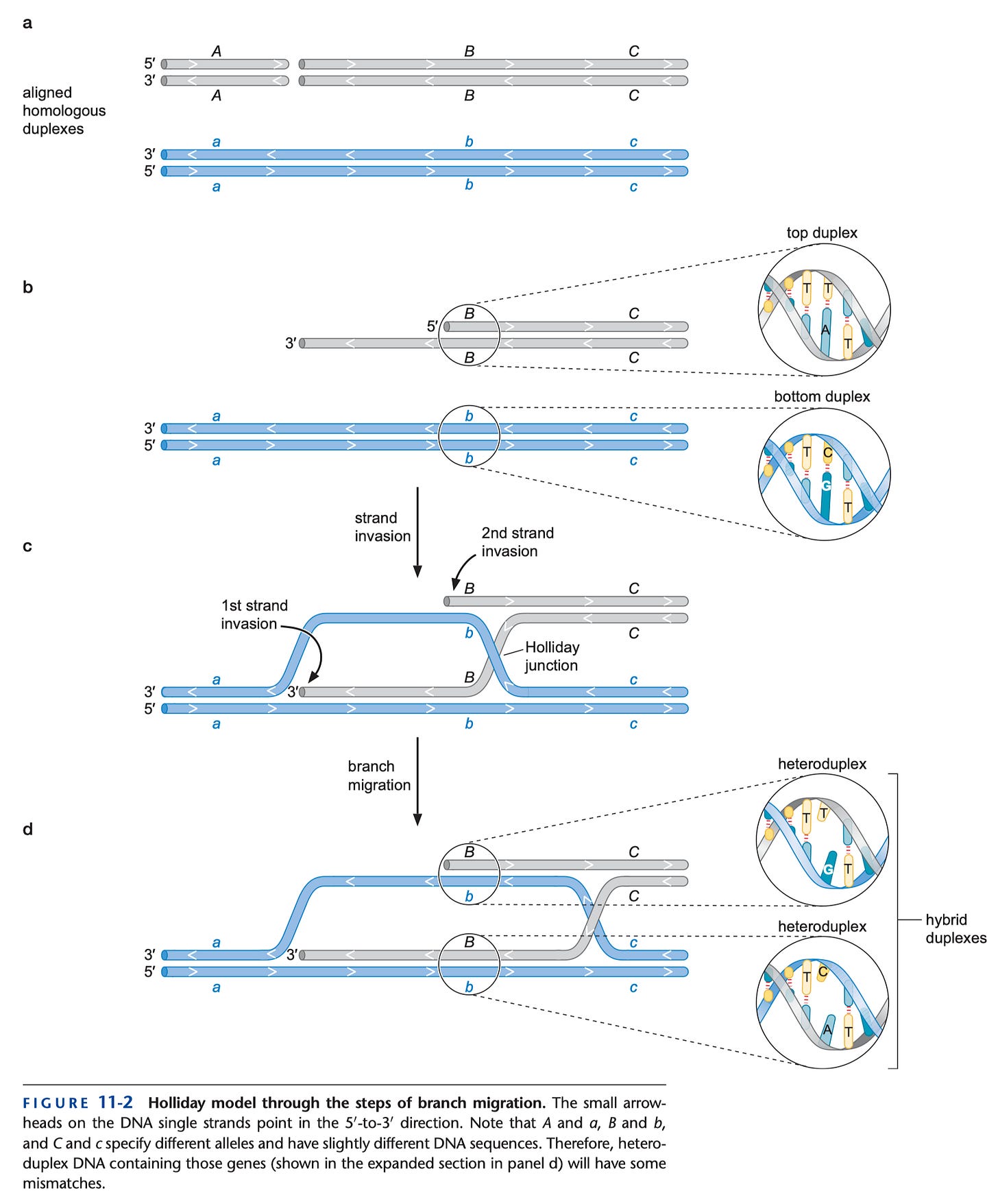
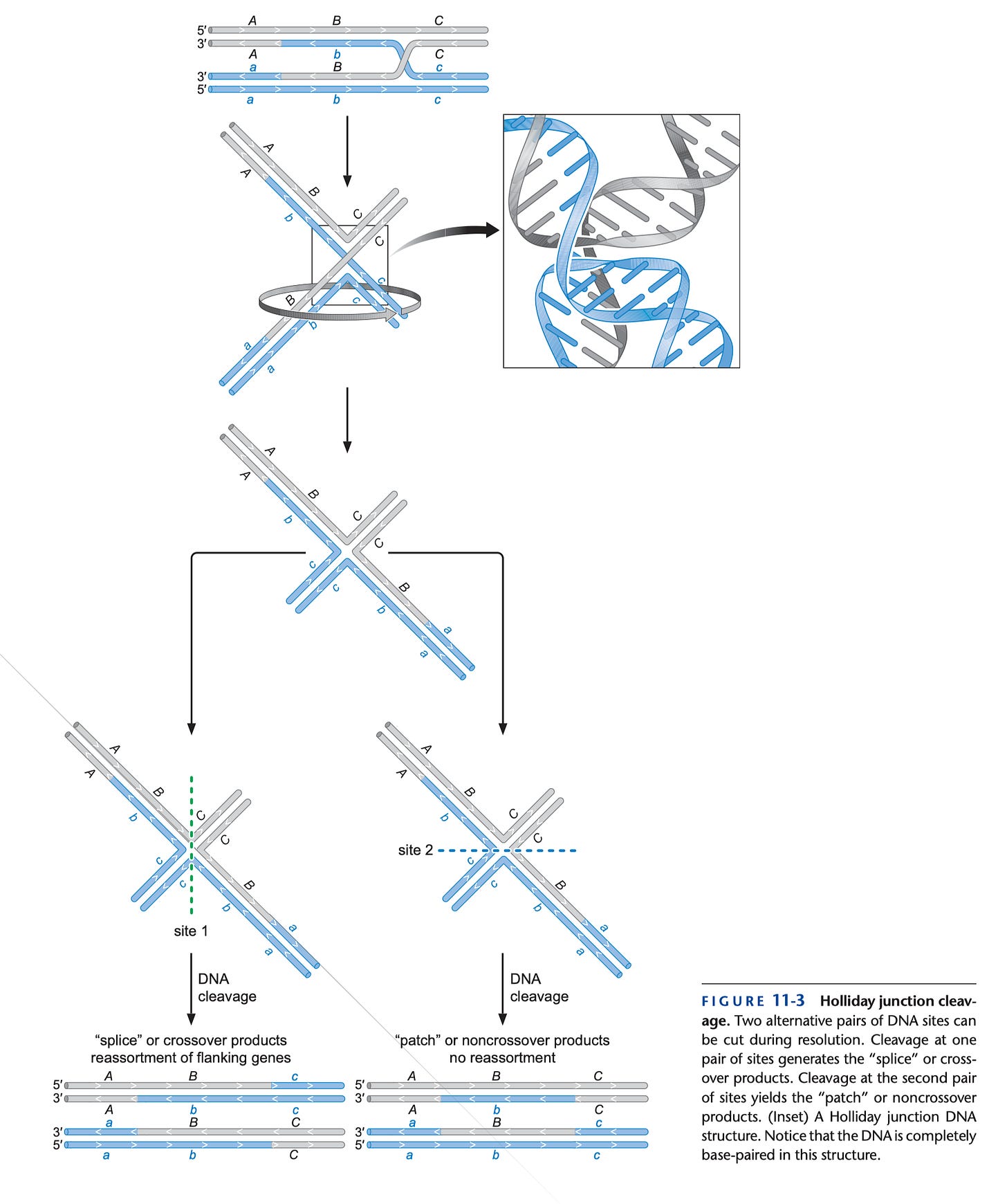
So, in principle, one might be able to regain antibody classes in a B cell that have been lost by recombining with the heavy chain locus at the other parental copy of chromosome (assuming that those constant regions are still there). However, homologous recombination is a much rarer pathway to repair the DNA than non-homologous end joining in general, and B cells use non-homologous end-joining in class switch recombination.
In some specific circumstances, IgG antibodies can cause mast cell activation and allergic reactions like anaphylaxis, but this is the exception rather than the rule. In particular, IgG-mediated anaphylaxis seems to involve a major role for neutrophils and platelets, which is very different from “standard“ allergic reactions.
In this instance I am referring specifically to type I Fc gamma receptors. I felt a discussion of type II receptors was not essential here.
For the purposes of this sentence, I am disregarding the inhibitory signaling role of CD32B.
An exception to this is possible if a bivalent IgG4 recognizes 2 different epitopes on the same antigen, but this would require a massive imbalance in the specificity of IgG4-secreting cells.
The ability of IgG antibodies to suppress inflammation or induce tolerance is a property that is driven by type II Fc gamma receptors and specifically the type I Fc gamma receptor CD32B (also known as FcγR2B). CD32B is unique because while it has a very strong resemblance on the outside to CD32A and CD32C, the internal part of the protein has a motif called an ITIM whereas the other type I receptors have ITAMs. This feature means that when IgG interacts with CD32B, it actively suppresses the signals from the IgG antibodies interacting with any other Fc gamma receptors in the cell. It is well understood that the presence of sialic acid on the glycan of IgG (including IgG4) is the key structural feature needed for this function, and this is not dependent on the antibody subclass. While all of the IgG subclasses can interact with CD32B, IgG4 does not have some kind of enhanced ability to engage this receptor relative to the other subclasses (in fact, just like with the other Fc receptors, it is reduced). Therefore, in most contexts, I would not be comfortable describing it as anti-inflammatory or tolerogenic because these are not active effects of IgG4. The anti-inflammatory consequences of IgG4 arise indirectly through its competition with other antibodies but the mere presence of IgG4 will not suppress pro-inflammatory signaling from other antibodies. IgG that bears sialic acid, in contrast, will.
If I could make a footnote within a footnote here though, even though in a vacuum sialylated IgG will suppress inflammation due to other IgG (or indeed any pathways that rely on ITAM signaling), this is not always the effect that is seen in the body. For example, CD32B is the only Fc receptor found on B cells (some people do have CD32C), and it has been shown that sialylated IgG raises the threshold for B cell participation in the immune response because CD32B reduces signaling through the B cell receptor when B cells engage with antigen. While in theory this sounds like a straightforward path to tolerance, the practical applications are broader. In fact, it has been shown that people who make sialylated IgG in response to the influenza vaccine tend to have better antibody responses and outcomes than those who do not.
So, what’s the conclusion here? A determination of tolerance has to be made empirically by observing what happens on exposure to the antigen. Even today with our vast knowledge of the immune system, the gold standard for determining food allergy is a double-blind, placebo-controlled food challenge, and not any marker that we measure.
While this statement as written is a true one, you can reasonably argue that the relative benefit (as compared with risk) of booster doses in some demographics is less clear than in others given a lower risk from COVID and a higher risk of concerning adverse events, such as teenage males with respect to myocarditis (although the latest data on this suggests this risk has declined markedly, supporting an effect of dosing interval- see slide 65). However, in someone who is unvaccinated the risks posed by COVID-19 are far greater than the risks posed by our vaccines, including the very young.
I felt that a discussion of the complement system was too vast for the scope of this post but it is probably worthwhile to give a very brief summary. In the body exist a number of proteins made in the liver known as complement, which have multiple roles. The complement proteins are made as inactive precursors which then have to be activated by environmental cues. The classical pathway of complement activation involves an antibody binding to the surface of a microbe and depositing complement proteins on it, helping the immune system to recognize and clear it. The complement system also results in formation of a pore structure called the membrane attack complex (MAC) which kills or inactivats microbes directly. In addition to these, there are the alternative and lectin pathways. Complement is a key component of protection against bacteria, especially encapsulated bacteria, with genetic defects in certain complement proteins often serving to make the infections deadly. Complement also has a critical role in supporting antibody responses. However, the complement system and the coagulation cascade, responsible for blood clotting, interact closely with one another, and so it is thought that complement activation can in some circumstances promote inappropriate clotting (along with other parts of the immune system).