SV40 Doesn't Cause Cancer (In Humans)
There isn't good evidence that SV40 causes cancer in humans, no matter how hard people try to panic monger about it. The concern is also moot because no one is being exposed to SV40 anymore.
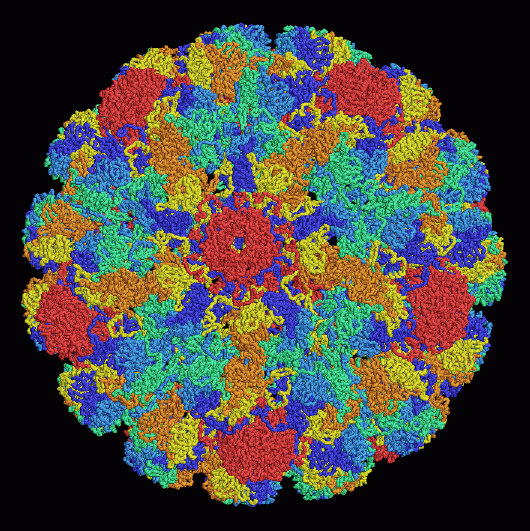
The gist: At present, bad-faith actors are attempting to foment a panic about the detection of an SV40 promoter sequence in the DNA used to make mRNA vaccines (a finding that is biologically meaningless for vaccinees even if we make many unjustified, charitable concessions about the work that flags this concern). The preprint has led some to insist that this vindicates claims about mRNA vaccines causing cancer because SV40 causes cancer in humans. Unfortunately for those people (and fortunately for the rest of us), no part of that is correct. In reality:
There is no increased risk of cancer seen from mRNA vaccines and “turbo cancer” doesn’t exist.
SV40 does not cause cancer in humans (details in this post).
An SV40 promoter (a sequence in the plasmid that is used to make the mRNA vaccines) is not the same thing as the T antigen of SV40, which is not the same thing as the SV40 virus.
There was indeed a period of justified concern about the ability of SV40 to cause cancer in humans, but large, well-done studies do not support a link. While SV40 can certainly cause cancer in some animals, the totality of the evidence to date does not support that it does in humans. For further explanation, I’m afraid you have to read the rest of the post.
Historical Context
To make (most) vaccines, you need the ability to make a lot of the microbe needed for the vaccine. In the case of viruses, this means you need cells that will faithfully replicate them in huge numbers. The issue here however is that most cells have defense mechanisms against viruses, which makes it hard to get enough virus from them. Nonetheless, there are cell lines that accomplish this quite well, including cells from the kidneys of rhesus macaques. In the 1950s and 1960s, this cell line was used to make the polio vaccine and a type of adenovirus vaccine. However, at the time, it was not known that this cell line was also infected with another virus known as simian virus 40 or SV40. SV40 also proved to be extremely hardy despite harsh environmental conditions: it retained some ability to replicate despite the same conditions that inactivated the polioviruses used in the polio vaccine.Sweet and Hilleman discovered the contamination in 1959, and by 1963, SV40 had been cleared from the vaccine supply, but an estimated 100 million people had been exposed through vaccination. Shortly after its identification, SV40 was discovered to have the ability to cause the formation of tumors in rodents and in cultured cells. This naturally prompted concern about whether SV40 could cause cancer in actual humans.
Basic Biology of SV40
For details, Fields Virology: DNA Viruses 7th Edition Chapter 1 on polyomaviruses is suggested and most information here is sourced from there unless otherwise indicated.
If you only care about how we know SV40 doesn’t cause cancer in humans, this section can be skipped.
To understand the validity of the concerns surrounding SV40, it is helpful to know a bit about the biology of SV40. SV40 is a type of DNA virus called a polyomavirus. Polyomaviruses are known to cause disease in humans, though mainly in immunocompromised individuals, with representative members of like BK, JC, and Merkel cell polyomavirus. The key piece of the biology of SV40 that enabled its ability to cause tumors (a process known as transformation) was a protein called the T antigen, specifically the large T antigen (LTAg), which we know because expression of LTAg in cells is enough to transform them even without the rest of the SV40 genome or virus (this is in fact how some cell lines were made). Note that the SV40 promoter has no capacity to do this; it can only tell RNA polymerases to generate an RNA sequence that lies directly downstream of the promoter.12
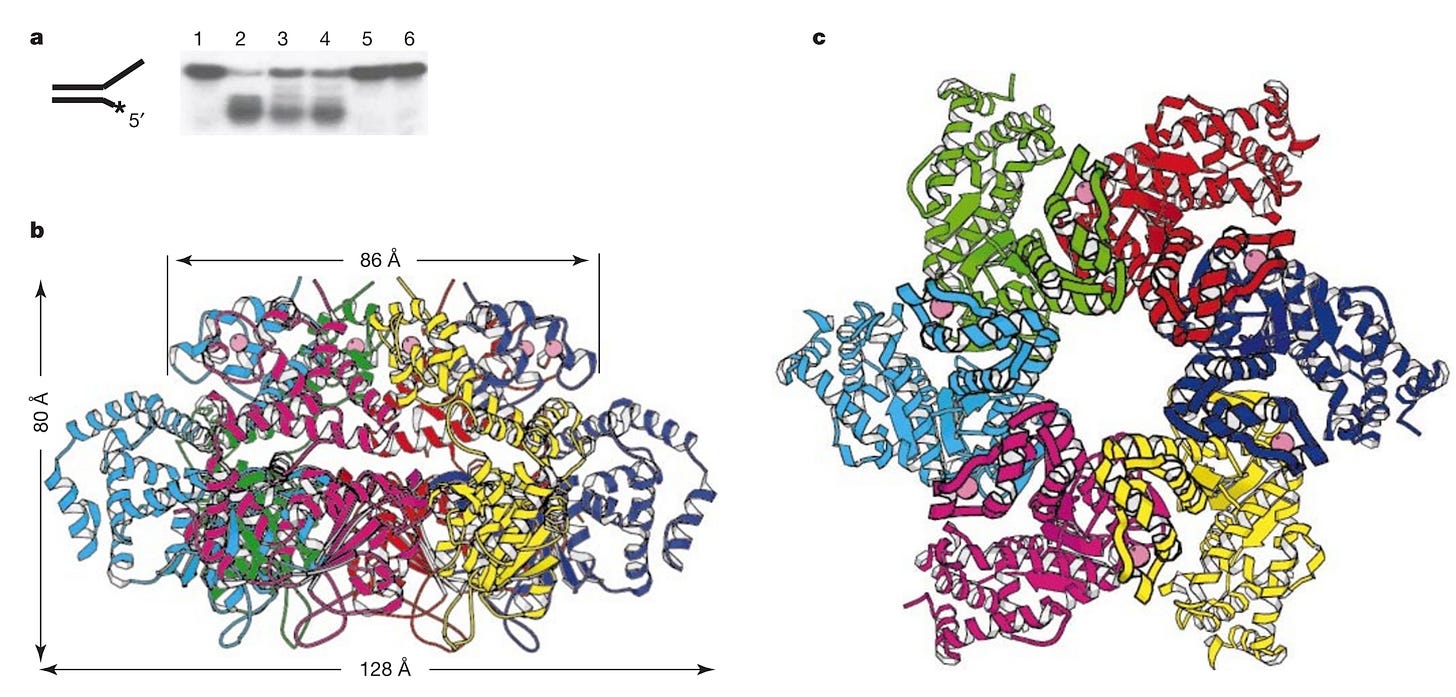
To understand how the T antigen works, we need to know the basics about how our cells divide. The cells of our body progress through a characteristic cycle with 4 major parts: G1, S, G2, and M. M (for mitosis- or meiosis in the case of sex cells) is the part of the cell cycle in which cells actually divide and make daughter cells. S (synthesis) is the part of the cell cycle in which cells copy all of their genetic material in preparation for dividing. Between S and M are the G1 and G2 phases, wherein G stands for “gap.” Some cells, such as muscle cells and neurons, enter a state known as G0 (sometimes called quiescence), in which they do not progress through the cell cycle for a very long time -potentially even decades- but this can be reversed under appropriate conditions. Some cells may also become senescent, wherein they are irreversibly trapped in G1 or G2. Throughout the cell cycle, there are checkpoints to ensure that the cell is prepared to progress into the next step:
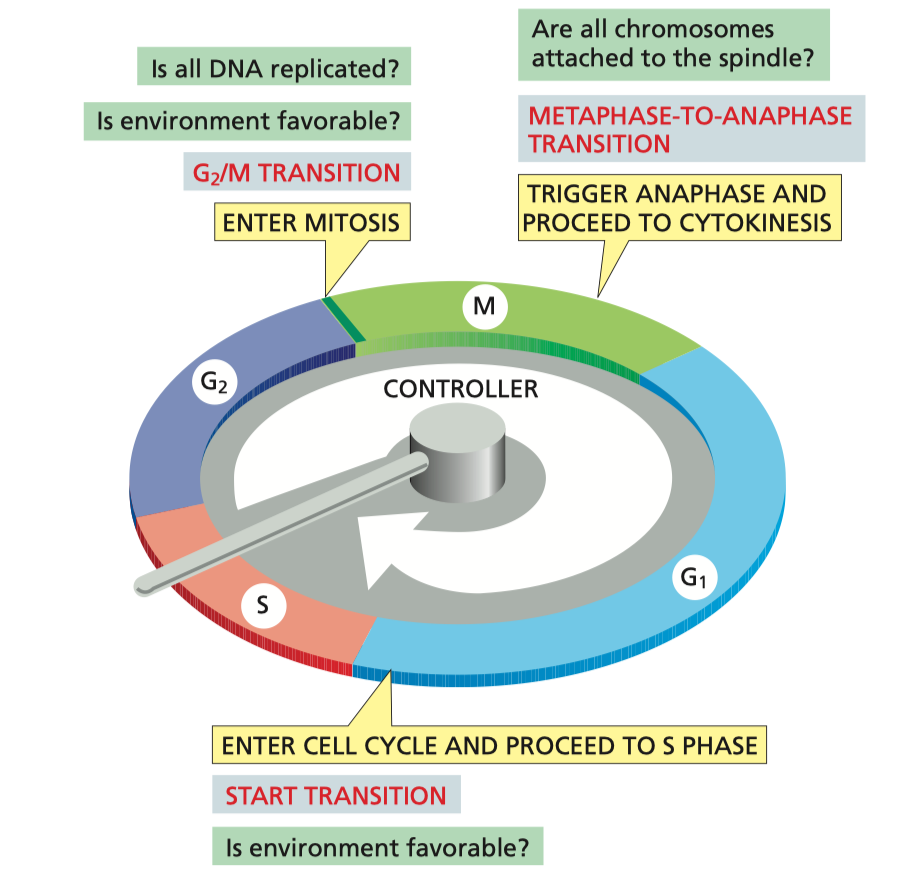
Proteins that promote progression through the cell cycle are known as proto-oncogenes and those that slow down progress are known as tumor suppressor genes.
The T antigen does a lot of things to cells that promote transformation, but for simplicity I will focus on the most important ones: LTAg has the ability to bind to and inactivate two proteins that are very important for regulating the cell cycle: p53 and retinoblastoma (Rb). Both of these proteins are tumor suppressor genes.
The effect of LTAg on the cell cycle is summarized below (the “┴” symbol indicates inhibition; arrows are dotted to emphasize that in the presence of LTAg, the normal stops directed by Rb and p53 do not occur).
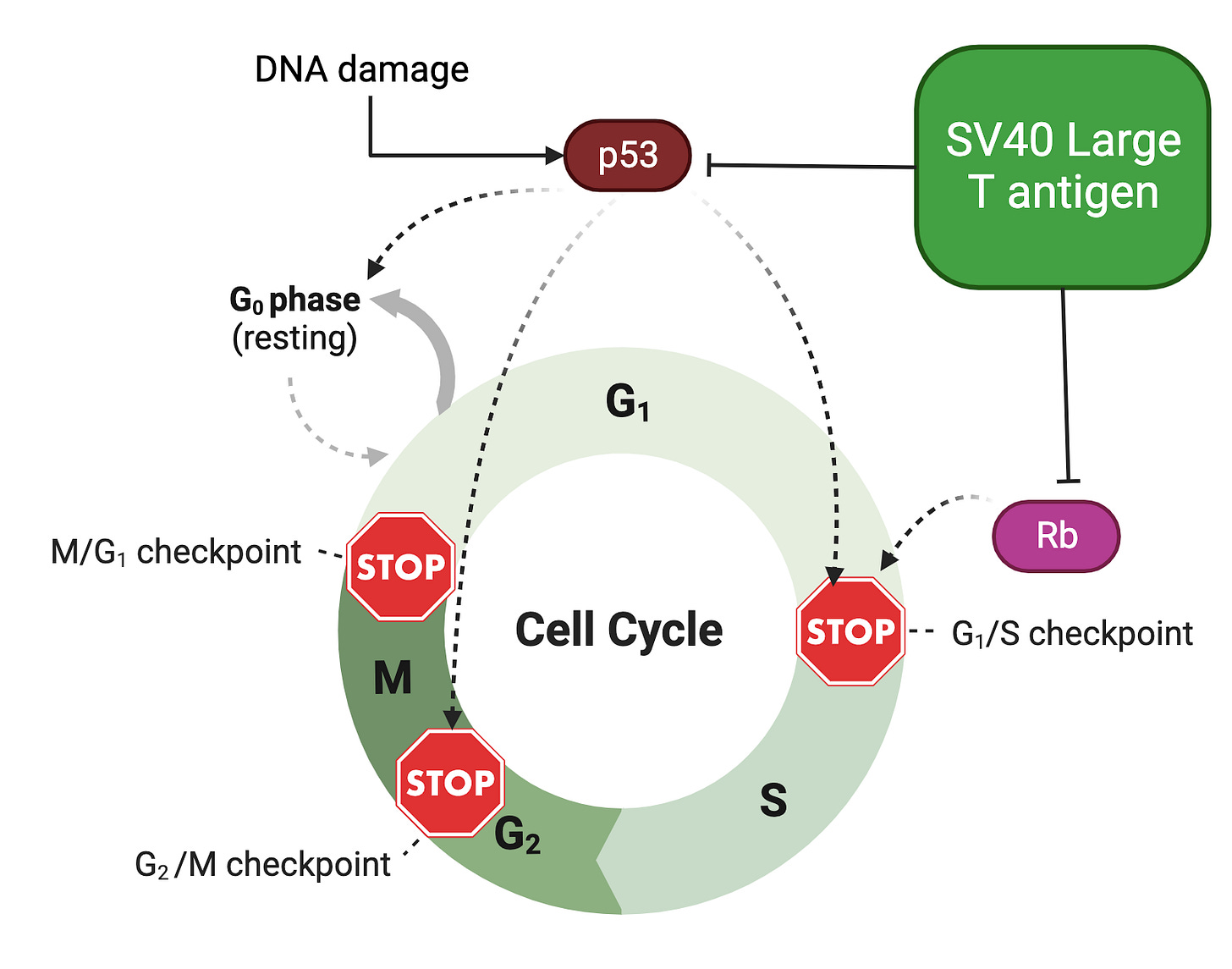
Retinoblastoma (Rb) encodes a protein that regulates transition into the S phase of the cell cycle (enabling copying of the DNA). When it is bound to the protein E2F, the cell cannot proceed into S phase. Normally, Rb detaches when it is phosphorylated (has phosphate groups attached to it), causing it to release E2F and allow transition into the cell cycle. Thus, if Rb is inactivated or unable to bind E2F in the first place, E2F will constantly drive progress into the S phase, and therefore replication of the DNA, even when it’s inappropriate.
p53 (sometimes called the “guardian angel of the genome”) is a protein that senses damage to the DNA and can either arrest progress through the cell cycle at multiple points until the damage is repaired or, if this fails, cause the cell to die by a pathway called apoptosis (sometimes described as cell suicide or programmed cell death). p53 can also cause cells to become senescent. In this way, cells infected with SV40, and specifically LTAg, progress through the cell cycle in an uncontrolled manner, regardless of the checkpoints that are ordinarily in place to prevent this.
In addition to large T, small T antigen also has roles in tumor formation in cells infected with SV40 but they are more minor. There is also a tiny T antigen, but its function is not clear. Other polyomaviruses may also have a middle T antigen, but SV40 does not.
Despite this, it should not be assumed that SV40 (or other polyomaviruses) automatically transform any cells they enter and cause uncontrolled tumor formation. SV40 is a virus with a lytic replication cycle, meaning that the cells it infects are ordinarily ruptured (lyse) and die. SV40 will form tumors only in cell types which are not permissive to lysis, but are permissive to transformation. Whether or not a cell can support transformation by SV40 tends to be very species specific. For example, SV40 cannot bind to a protein called primase in rodent cells. Primase carries out an essential part of DNA replication. For this reason, SV40 is not able to replicate within rodent cells, and thus not enough virus is produced to rupture the cells, but T antigen is still present, so they are transformed. Additionally, though p53 is a target of the T antigen, the levels of p53 in a cell at baseline can affect a cell’s susceptibility to transformation. Higher levels of p53 make it more difficult to transform a cell by SV40 (and other polyomaviruses), and the amount of p53 can vary by tissue and cell type.
SV40 and Human Cancer
The IARC (International Agency for Research on Cancer) monograph has an excellent summary of the evidence regarding the role of SV40 in human cancer. Broadly, the conclusion from this evidence is that SV40 is not shown to cause cancer in humans. However, if you look at older sources, you might not get the same answer. This is because major problems were detected with many pieces of older evidence that falsely implicated SV40, resulting in incorrect conclusions about the risk it posed to humans (details below). To summarize the investigations (in no particular order):
Serological studies: These looked for the presence of antibodies against SV40. Unfortunately, there’s a serious problem with these investigations: SV40 is very structurally similar to other polyomaviruses which infect humans (and are far more common). When the antibodies were depleted of those that bound these other polyomaviruses, the reactivity of the samples to SV40 almost completely vanishes, making it unlikely that exposure to SV40 ever occurred and suggesting that the antibodies were made in response to other polyomaviruses (which humans are much more likely to be exposed to).
In vitro studies of human cells: These studies examined whether or not SV40 could transform cells or at least establish a persistent infection in them. These studies gave mixed results and it remains controversial whether or not SV40 can establish a persistent infection in human cells or cause tumors to form (but this does vary by cell type).
Animal Studies: Animal studies such as those in hamsters supported that SV40 could cause tumors to develop, although these results were not universal. For example, in one study of white mice inoculated with SV40 (albeit without controls), no tumors developed in any of the mice. Thus we cannot say that just because SV40 causes tumors in one animal means it will cause it in all animals exposed to SV40.
Presence of SV40 within tumors: These studies are some of the most fraught. Some studies were able to detect SV40 DNA in human tumors, but the SV40 sequences often appeared fragmented, and were low in copy number which is inconsistent with knowledge of what would be required for transformation. Later evidence suggested that these investigations actually contaminated the samples with plasmids (circular DNA not associated with chromosomes) that contain SV40 sequences, as these are very common molecular biology reagents.
Epidemiological Studies: These studies are by far the most important in investigating whether or not exposure to something causes cancer. Many things can happen in a petri dish and in animals, but if they don’t lead to an effect in actual humans, they are not relevant. Broadly, these all ask whether or not those who had been exposed to SV40 had a higher risk of cancer or death than those who had not using different methods. Consistently, these fail to find a difference in the risk of cancer or death between exposed and unexposed individuals, suggesting that SV40 does not cause cancer in humans.
Given these findings, IARC writes (emphasis mine):
There is strong and consistent evidence from animal and cell-culture studies that SV40 can be directly oncogenic and transforming through its oncoproteins, i.e. the LTs encoded in the early region of its genome. The mechanisms involve immortalization, transformation, and inhibition of apoptosis. There is only weak and controversial evidence for such mechanisms being active in human tumours.
The presence of SV40 DNA, based on analysis by PCR, has been reported in a broad variety of human tumours by several groups but not by others. Experimentally substantiated doubts have been raised that laboratory contaminations with commercial plasmids may have contributed to some positive PCR results. Other differences in experimental methods may also contribute to discrepancies across laboratories.
…
Millions of people were exposed to SV40-contaminated vaccines, but multiple follow-up studies of recipients of contaminated inactivated poliovirus vaccines have not revealed them to be at increased risk of cancer compared with unexposed cohorts.
….
There is inadequate evidence in humans for the carcinogenicity of SV40.
…
SV40 is not classifiable as to its carcinogenicity to humans (Group 3).
For context, IARC is an extremely conservative expert body when it comes to the risk of cancer, and group 3 is the lowest risk category they offer. Notably, IARC does not categorize substances by the likelihood that exposure to the substance will cause cancer but the certainty of the evidence that it does, which creates confusion.
Actions Taken to Prevent SV40-like Incidents Again
While it is fortunate that SV40 does not appear to be associated with health problems in humans, the possibility that a cell strain used for vaccine production could contain something harmful to humans cast a large shadow and prompted major changes in how vaccines were produced. One of these changes was the use of human cells in the production of vaccines, and specifically, fetal cells. These have major advantages over other types of cells for vaccine production, which I have previously written about here. It should be noted that vaccines do not contain any aborted fetal tissues, the incredible dividing capacity of these cells means that we do not require an abortion-to-vaccine pipeline to constantly supply vaccine manufacturers with cell strains, and only a few vaccines use cell strains from fetal tissues.
In addition to these, one of the many advantages of newer technologies for making vaccines is that you can get around needing cell lines or cell strains at all. mRNA vaccines for example do not require anything other than the DNA sequence for the mRNA and the machinery to construct new mRNA (as well as the fats used in the lipid nanoparticle that allow for delivery). This means that you can make mRNA vaccines entirely outside of cells, and thus there’s no potential to contaminate them with an unknown human virus. The only step requiring the use of cells is when the DNA for the mRNA vaccine is being replicated- this is done by inserting the DNA into bacteria and growing that bacteria to a suitably high level (wherein they will replicate the DNA containing the mRNA vaccine sequence) before killing them and recovering the DNA. By using bacteria, you avoid the potential for contamination by viruses that can infect humans. Obviously, this DNA is purified out and any residuals from the process of cloning the plasmid are removed.
Beyond these, the manufacturing process now contains extensive purity testing long before vaccines are ever released to the public. The specific type of purity test depends on the vaccine in question. For example, some vaccines contain only a single protein from a microbe. Proteins can be separated and examined through a process called SDS-PAGE, wherein each protein produces characteristic bands. By the time the vaccine has been produced, the only bands that appear on SDS-PAGE should be those corresponding to the vaccine antigen. Vaccines which require the use of replicating virus during production are subjected to extensive DNA sequencing to detect any adventitious agents (which would readily detect the presence of a virus like SV40).
SV40 is not present in any vaccines given today, including mRNA vaccines.
Conclusion
In the 1950s and 1960s, there was reasonable concern that contamination of cell strains used to make vaccines by simian virus 40 (SV40) could result in human cancers, as SV40 had been shown to transform various cells and cause tumors in animals. These contaminated vaccines were rapidly removed from the market and many investigations were conducted. Epidemiological studies looking at the risk of cancer in those who received contaminated vaccines and those who had not consistently failed to demonstrate an increase in the risk of cancer among SV40-exposed individuals. However, laboratory investigations of tumors and antibodies to SV40 initially suggested the presence of the virus. It later became apparent however that the genetic sequences of SV40 that had been detected were likely the results of contamination, and the antibodies to SV40 were actually directed against other polyomaviruses humans had been exposed to. Taken together, the evidence does not support that SV40 can cause cancer in humans.
For those interested: to make the Pfizer/BioNTech vaccine, one first needs many copies of DNA that encode the spike protein mRNA. To do this, a circular piece of DNA called a plasmid is inserted into bacteria which are cultured to a high level. The plasmid contains the gene for the spike protein and a gene called KanR, which renders these bacteria resistant to the antibiotic kanamycin. The KanR gene’s expression in the bacteria is controlled by an SV40 promoter, although if McKernan’s work is to be believed, this is redundant because directly upstream of the SV40 promoter is an AmpR promoter. The specific promoter is not relevant beyond that different cells may recognize different promoters with different efficiency, and if you choose one that the cell in question cannot recognize when designing your plasmid, you will not get expression of that plasmid’s gene(s).
The point of this in production is that this allows you to select only those bacteria that have taken up and replicated the plasmid by growing them in the presence of kanamycin. Once the bacteria have replicated to a sufficiently high level, copying the plasmid at each step as well, the bacteria are lysed and the plasmid is recovered and purified (i.e. no bacterial proteins, endotoxin, or kanamycin makes it past this stage). From here, the plasmid is cut to be made linear because the gene for the spike protein does not have a transcription stop site (if you didn’t cut it, the RNA polymerase would endlessly loop around the plasmid). You then add T7 polymerase (because the spike gene is under control of the T7 promoter and T7 is very specific for the T7 promoter) and the nucleotides for the mRNA and can generate many copies of spike mRNA. These mRNAs are again purified out to separate them from the DNA with the aid of an enzyme that destroys DNA and are then packaged into lipid nanoparticles, at which point they are ready for release (once all the quality control testing from the lot has been passed).
An analogous process is used to make the Moderna mRNA vaccine, but the specific details of the process and sequences involved are not public; they are only “public” in this case because confidential EMA documents describing these details were hacked in a cyberattack and released.
There has been some discussion about the ability of the SV40 promoter to direct nuclear import of DNA in the context of mRNA vaccines and the suggestion that this represents a concern. As a reminder, there may be trace residual quantities of short, fragmented DNA within mRNA vaccines but regulators ensure that these are far below extremely conservative limits (which arguably would not even apply here because they come from investigations where DNA from transformed cells is introduced into healthy ones, not plasmid DNA that lacks any transforming sequences). SV40’s promoter can indeed help enable sequences to enter the nucleus but this is not relevant here for several reasons.
First and most obviously: the sequences in question would have to be intact. During the production of mRNA vaccines, the product is treated with an enzyme that destroys DNA called DNase I. This drastically reduces the likelihood of any fragment containing a functional SV40 promoter.
In addition to this, we now understand in great detail that nucleic acids like RNA and DNA need to meet certain quality control measures within our cells, which include things like the modifications that are applied to them and where they are in the cell. Our cells’ cytosols are filled with enzymes that destroy DNA because DNA is almost never supposed to be in the cytosol. When SV40 enters cells, it is entering in the form of a viral particle whose genome is shielded from those enzymes and it goes to the nucleus- a situation that does not apply here because mRNA vaccines can only deliver their cargo into the cytosol.
Furthermore, if somehow this fails to destroy the DNA in question, the cell will initiate a suite of antiviral defense mechanisms that ultimately end in the cell dying.
Additionally, if every single pathway involved in sensing foreign DNA still somehow fails, the cell will die regardless because it will be made to express the spike protein and will therefore be killed by the immune response against spike.